Courses taught by Professor Marc Hodes
Professor Hodes teaches many courses at Tufts University, many related to Transport Phenomena as per the following list.
ES 08 Fluid Mechanics (now part of ME 50-51 sequence)

An introduction to fluids at rest and in motion. Fluid properties. Pressure and velocity variations in flows. Mass, momentum, and energy conservation in a flowing fluid. Bernoulli’s equation and inviscid flows. An introduction to viscous flows. Dimensional analysis. Drag and lift of moving objects. Fall.
ME 16 Heat Transfer (now part of ME 50-51 sequence)

Steady-state and transient conduction in solids; numerical solution of conduction problems; radiative heat transfer; forced and natural convection. Introduction to boiling and condensation heat transfer. Heat exchanger analysis.
ME 20 Mechanics I
Analysis and problem-solving in statics. Vector and matrix analysis. Force-moment balance equations. Analysis of stress and strain. Behavior of isotropic materials. Area moments of inertia. Behavior of members subjected to axial, torsional, and flexural loadings, combined stress, compression members, and columns.
ME 100 Applied Mathematics for Engineers
Review of ordinary differential equations and oscillatory phenomena. Fourier series and applications. Orthogonal functions, Bessel functions. Partial differential equations and their applications to fluid mechanics, heat transfer, vibration and wave propagation.
ME 150 Therm-Fluid Transport I
Advanced topics in fluid mechanics. Viscous and inviscid flows. Strain rate, vorticity and streamline coordinates. Differential conservation laws for mass, momentum, and energy. Dimensional analysis. Lubrication flows. Momentum and thermal laminar boundary layers. Laminar-turbulent transition. Reynolds stress and turbulence modeling. Turbulent boundary layers. Flow modeling.
ME 151 Therm-Fluid Transport II
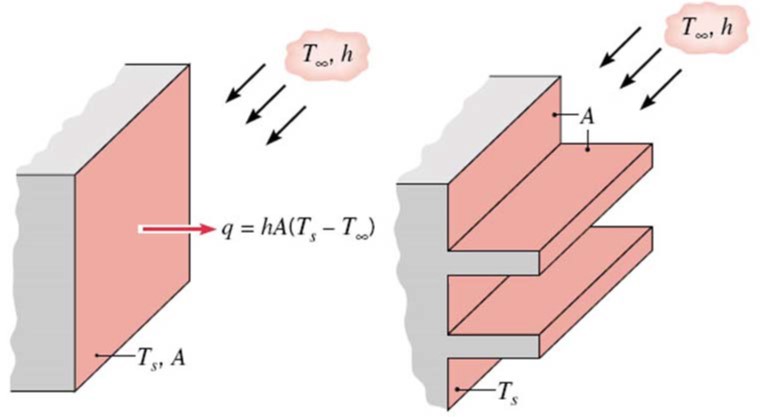
Advanced topics in heat transfer. Multi-dimensional conduction. Transient conduction including moving boundary problems. External forced and natural convection. Internal forced and natural convection. Developing flows and transition to turbulence. Condensation and boiling heat transfer. Radiation and conjugate heat transfer involving radiation. Temperature and heat flux measurements. Numerical techniques.
ME 158 Thermal Management of Electronics
This course provides the know-how to design hardware to control the temperature of electronic and photonic components. The dependence of component reliability on operating temperature is addressed and thermal management challenges at the die, package, circuit board, shelf, cabinet, room, and environmental levels are considered. The core of the course concerns the design and analysis of key enabling technologies, i.e., heat spreaders, thermal interface materials (TIMs), thermoelectric modules (TEMs), heat pipes, and heat sinks. Selected emerging technologies, e.g., microchannel cooling and transient thermal management of portable consumer electronics are introduced and the design of energy-efficient thermal management solutions is considered.
ME 193 Analytical Transport Phenomena
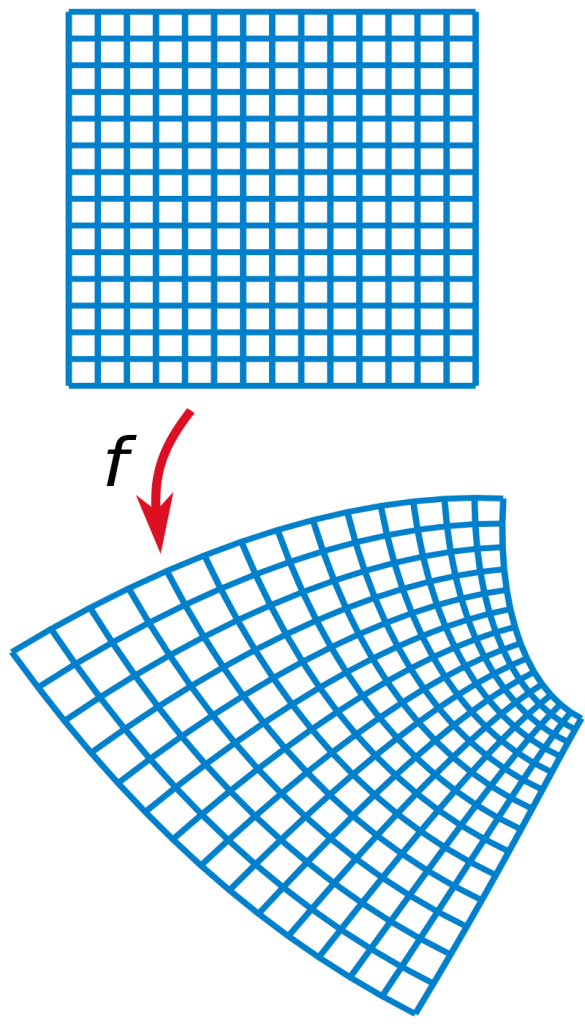
Analytical methods for the solution of fluid mechanics and heat and mass transfer problems. Convective transfer equations and associated boundary conditions. Perturbation methods and complex analysis and their application to representative transport phenomena problems. Introduction to the numerical solution of partial differential equations using the Chebyshev collocation (spectral) method.
Course taught by Professor Georgios Karamanis
Professor Georgios Karamanis offers this practical course for students who are interested in modeling and simulation with commercial software.
ME 193 Simulation for Mechanical Engineers
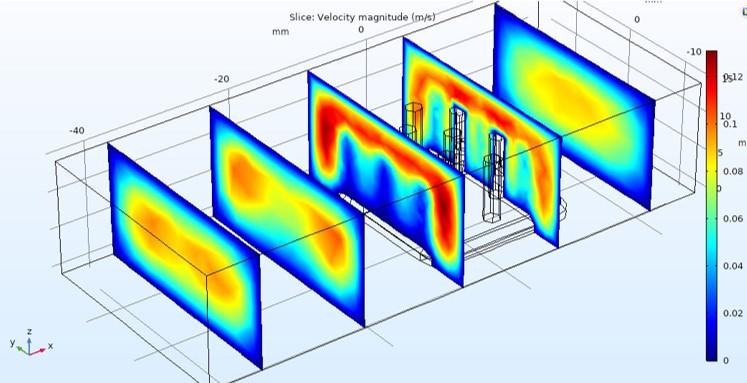
This course provides the know-how to perform structural, thermal, and fluid flow analysis using commercial software packages (SOLIDWORKS, COMSOL, ANSYS Workbench, MATLAB). Students learn the modeling workflow for each type of simulation and how to select appropriate model properties, boundary conditions, and numerical algorithms based on the underlying physics. Students also learn how to interpret simulation results and identify sources of error.