By Dr. Vicky Yang, Revised from original syllabus by Dr. Barret Bulmer
OBJECTIVES for CV RESPONSE TO PULMONARY HYPERTENSION
- Know the definition of pulmonary hypertension and the normal intracardiac, pulmonary arterial and aortic pressures
- Know the five main groups of pulmonary hypertension as defined by the World Health Organization
- Know the systems affected by and clinical features of pulmonary hypertension
- Understand how pulmonary arterial pressure can be determined based on the Doppler velocity of tricuspid regurgitation
- Know the treatment options available for the treatment of pulmonary hypertension
Pulmonary Hypertension
Pulmonary hypertension (PHT) is present when the mean pulmonary arterial pressure is greater than 25 mm Hg.Mean pulmonary arterial pressure (MPAP) is related to the pulmonary vascular resistance (PVR) and pulmonary blood flow (PBF) as shown in the equation:
MPAP = (PVR × PBF) + mean pulmonary capillary wedge pressure
A classification scheme for PHT devised on the basis of mechanisms was introduced by the World HealthOrganization. The groups, with examples of associated veterinary conditions, are as follows:
- Group I: Pulmonary arterial hypertension
- Idiopathic
- PHT associated with systemic to pulmonary (left-to-right) shunts
- PHT associated with portal hypertension
- PHT associated with drugs/toxins
- PHT associated with heartworm infection
- Group II: Pulmonary venous hypertension
- Mitral valve insufficiency/mitral stenosis
- Myocardial disease with elevated left ventricular diastolic pressure
- Secondary to congestive heart failure
- Group III: PHT associated with chronic respiratory disease/hypoxemia
- Chronic obstructive pulmonary disease
- Interstitial lung disease
- Chronic exposure to high altitude
- Group IV: PHT associated with chronic pulmonary thromboembolism (or worm embolism)
- Group V: PHT due to miscellaneous disorders directly affecting the pulmonary vasculature
- Heartworms (Dirofilaria immitis) (*newly classified into Group I and IV)
- Angiostrongylus vasorum
ETIOLOGY / PATHOPHYSIOLOGY
- The principal vascular changes that produce pulmonary hypertension include vasoconstriction, smooth-muscle cell and endothelial cell proliferation, and thrombosis. These processes reduce the cross-sectional area of the pulmonary arterial bed and the pulmonary compliance, thereby increasing pulmonary vascular resistance.
- Pulmonary endothelial cell dysfunction or injury likely contribute to these homeostatic imbalances viaalteration of the complex interactions between various vasodilators (e.g., prostacyclin, nitric oxide) and vasoconstrictors (e.g., thromboxane A2, endothelin-1, serotonin), growth inhibitors and mitogenic factors, and antithrombotic and prothrombotic determinants.
- Pulmonary venous hypertension secondary to myocardial disease in cats and myocardial and valvular disease in dogs may contribute to increased pulmonary arterial pressures via retrograde transmission of left atrial pressure to the pulmonary vascular bed, hypoxia-induced vasoconstriction of the pulmonary arteries, endothelial dysfunction and vascular remodeling.
- Animals with PHT secondary to chronic respiratory disease develop elevated pulmonary arterial pressure as a result from direct damage from the primary disorder, hypoxia- induced vasoconstriction and vascular remodeling.
Systems Affected
- Respiratory:
- Coughing, respiratory distress, and tachypnea may manifest as a result of any primary underlying pulmonary disease.
- Vascular remodeling and hypoxemia associated with PHT may also independently reduce lung compliance and contribute to respiratory compromise.
- Cardiovascular:
- Increased pulmonary arterial pressure contributes to right ventricular concentric hypertrophy in an effort to normalize ventricular wall stress.
- Depending on the speed with which it develops and its severity, pulmonary hypertension mayultimately increase right ventricular end-diastolic and right atrial pressures producing right-sided heart failure.
- Exertional syncope may occur secondary to hypoxemia, exercise-induced vasodilation and aninability to increase cardiac output/pulmonary blood flow, arrhythmias or reflex-induced bradycardia, and hypotension.
- Musculoskeletal: Hypoxemia, ventilation-perfusion mismatch, lactic acidosis and fixed cardiac output contribute to exercise intolerance and possibly weakness.
- Nervous: Hypoxemia may contribute to depression and lethargy, or when combined with arrhythmias or activity may contribute to syncope.
- Hepatobiliary: Concurrent right-sided congestion may contribute to cholestasis.
SIGNALMENT / HISTORY
- The signalment varies greatly based on the etiology that produced the PHT. Population characteristics from two large retrospective studies identified an age range from 2 months to 17 years, although the largest percentages of dogs in both studies were > 10 years of age. Similarly, there is a wide weight distribution. There is no apparent sex predilection, and it isdifficult to predict if there are breed predispositions, although:
- dogs predisposed to degenerative mitral valve disease will be at increased risk for developing pulmonary venous hypertension
- geographic distribution and heartworm preventative status may increase the likelihood of PHT associated with heartworm disease
- West Highland White Terriers with interstitial pulmonary fibrosis may also be at higher risk for development of PHT.
- Depending on the etiology, severity, and rate of onset of the PHT, owners may report a slow, insidious onset of clinical signs or an acute, rapid-onset of life-threatening respiratory compromise.
Risk Factors
- Conditions that contribute to hypercoagulable states (e.g., protein losing nephropathy, hyperadrenocorticism, neoplasia, immune-mediated hemolytic anemia) may increase the risk for pulmonary thromboembolism and acute development of PHT.
- Acute development of PHT secondary to embolization of Dirofilaria immitis may occur following adulticide therapy.
- Large left-to-right congenital cardiac shunts may contribute to increased pulmonary blood flow and vascular remodeling, both of which contribute to PHT.
- Although it is generally accepted that dogs have low pulmonary vascular reactivity, there is some risk ofdeveloping mild to moderate PHT resulting from chronic hypoxemia associated with living at high altitude.
Historical Findings
- Exercise intolerance
- Coughing, tachypnea, dyspnea
- Syncope
- Abdominal distension/right-sided heart failure
CLINICAL FEATURES
- Coughing, tachypnea, dyspnea, or orthopnea
- There may be audible crackles and wheezes during pulmonary auscultation
- Murmurs of tricuspid or pulmonic insufficiency may be auscultated
- A split S2 associated with delayed pulmonary valve closure may be audible
- Arrhythmias may be audible
- Evidence of right-sided heart failure may be present including jugular venous distension, positive hepatojugular reflux, hepatomegaly and/or ascites
- Cyanosis may be evident in severely compromised animals
- There may be an audible murmur or gallop correlating with the underlying etiology (e.g., left apical systolic murmur associated with mitral valve insufficiency or a left basilar continuous murmur associated with a left to right shunting PDA)
DIAGNOSTICS
- Animals with severe PHT may be in extremely critical condition, and the diagnostic tests performed must be dictated by the clinical situation.
- Thoracic radiographs
- Although thoracic radiographs are insensitive for identification of PHT, they are useful for evaluation of underlying pulmonary disease.
- In severe cases of PHT, dilation of the main pulmonary artery and right heart enlargement may be identified.
- Thoracic radiographs
- An enlarged caudal vena cava, ascites, hepatomegaly, and in some cases, pleural effusion may accompany right-sided heart failure.
- Left atrial and left ventricular enlargement with pulmonary venous congestion may accompany left-sided heart disease that produces PHT.
- Dilated, tortuous, and truncated pulmonary arteries may be seen with heartworm disease.
- Peripheral pulmonary arterial markings may abruptly stop with pulmonary thromboembolism.
- Electrocardiography
- Although electrocardiography is not able to diagnose PHT, it may identify patterns of right ventricular enlargement, ST segment depression, or arrhythmias that warrant institution of antiarrhythmics.
- Echocardiography
- Two-dimensional, spectral and color-flow Doppler echocardiography often allows for the non-invasive assessment of PHT.
- Right ventricular concentric and eccentric hypertrophy may be identified in cases of moderate to severe PHT in response to increased afterload, elevated end- diastolic pressures, and valvular insufficiencies.
- Interventricular septal flattening may be identified when right ventricular pressure exceeds that of left ventricle.
- Dilation of the pulmonary artery and main branches may be present.
- Small end-diastolic left ventricular diameters may be identified resulting from reduced leftventricular preload in cases of severe PHT. This may also contribute to systolic anterior motion of the mitral valve.
- Pulmonic stenosis or other complex defects of the right ventricular outflow tract can be ruled out as etiologies for the right ventricular hypertrophy.
- The presence of tricuspid insufficiency or pulmonic insufficiency enables estimation of pulmonaryarterial pressure with the use of the modified Bernoulli equation:
Pressure gradient = 4 × velocity2
- Echocardiography enables identification of concurrent mitral valve insufficiency, myocardial disease, or congenital heart disease that may contribute to PHT.
- Nuclear imaging and computerized tomography may be useful for identification of large pulmonary thromboemboli.
- Cardiac catheterization
- Although infrequently performed, placement of a Swan-Ganz catheter to measure pulmonary arterial pressures, estimate left atrial pressure, perform thermodilution measures of cardiacoutput and calculate pulmonary vascular resistance may be considered the gold standard for the diagnosis of PHT.
- Direct pulmonary arterial measures are further used in humans to assess the acute vasoreactivityof the pulmonary arteries to short-acting vasodilators and enable identification of candidates for chronic oral vasodilator therapy.
- Pulmonary angiography may help identify large pulmonary thromboemboli, although life-threatening complications have been reported in people with severe, acute PHT.
- A complete blood count, biochemical profile, urinalysis and heartworm serology should be performed toassess for underlying disease conditions that may contribute to PHT or hypercoagulability. These diagnostics also allow for investigation of consequences that may be attributable to the reduced cardiac output that frequently accompanies acute, severe PHT.
Pathologic Findings
- Dogs with PHT have been reported to have the same constrictive and complex lesions as those identified in people including four main histologic subsets:
- isolated medial hypertrophy
- medial hypertrophy-intimal thickening without plexiform lesion
- variations of the plexiform lesion and medial hypertrophy-intimal thickening was the most common pattern in one study
- isolated arteritis
- Pulmonary and cardiac lesions vary markedly in animals with primary lung or heart disease, pulmonary thromboembolism, and heartworm disease.
THERAPEUTICS
- The therapeutic objectives for the management of PHT would ideally include
- resolution of any underlying pulmonary disease or complicating factor contributing to the elevated pulmonary arterial pressure, and
- therapeutics aimed at reducing the pulmonary arterial pressure
- Unfortunately many of the primary pulmonary diseases are severe and irreversible by the time PHT is diagnosed.
- Similarly, although vasodilatory agents would seem beneficial, they may actually be contraindicated in the face of PHT related to pulmonary disease as they could exacerbate V/Q mismatch by dilating areas that are not ventilated.
- Further complicating the use of vasodilatory agents (e.g., amlodipine) is the fact that most traditionalarterial vasodilators more effectively reduce systemic arterial pressures than pulmonary arterial pressures and may produce symptomatic hypotension in the face of fixed right ventricular output.
- Many of the new and potentially promising vasodilatory agents used in people, including endothelin receptor antagonists and constant rate infusion, subcutaneous and oral synthetic prostacyclin analogs are often cost-prohibitive or difficult to administer in veterinary species.
Drug(s) of Choice
- Oxygen
- Although dogs are generally accepted to have low pulmonary vascular reactivity, oxygen supplementation may be useful at reducing hypoxic induced arterial vasoconstriction.
- Sildenafil
- A phosphodiesterase V inhibitor
- There are varying reports regarding its ability to reduce echocardiographically determined pulmonary arterial pressure
- There are no studies that clearly define the appropriate dose of sildenafil for the management of PHT in dogs, but we typically start with 1-2mg/kg q8 hours
- Diuretics, ACE inhibitors and positive inotropes are used as indicated to treat valvular disease ormyocardial disease that is contributing to PHT, or left- and right-sided heart failure.
- Abdominocentesis or thoracocentesis may need to be performed in animals compromised by ascites and pleural effusion, respectively.
- Atrial septostomy has been reported to improve hemodynamics and clinical signs in some humans with pulmonary arterial hypertension.
- Therapy in humans is usually palliative in an effort to prolong survival until a heart-lung transplantation can be performed.
Expected Course and Prognosis
- The expected course and prognosis for animals with PHT are difficult to define because there are many etiologies that contribute to the disease.
- Overall, with the exception of heartworm disease with resolution or reduction of PHT following adulticidetherapy, the prognosis for animals with moderate to severe PHT is guarded.
- In most cases where PHT is secondary to chronic respiratory disease, the underlying pulmonary pathology is severe and irreversible by the time PHT is diagnosed.
- Animals with mild to moderate PHT secondary to valvular disease or myocardial disease have a fair short-term prognosis and guarded long-term prognosis assuming the disease is progressive.
OBJECTIVES for CV RESPONSE TO SYSTEMIC HYPERTENSION
- Know the two primary determinants of blood pressure
- Know the four most potent vasoconstrictors
- Know the two vasodilatory substances
- Know the two most common systemic disease processes that contribute to hypertension in the dog and cat
- Know the target organs for systemic hypertension and the changes that may occur within each system
Systemic Hypertension
Blood pressure is defined as the force exerted by the blood against any unit area of the vessel wall. Most commonly this pressure is described by mmHg. Frequently, systemic venous pressures are measured utilizing a column of water, where 1 mmHg is equal to 1.36 cm of water.
The two primary determinants of blood pressure (BP) are cardiac output (CO) and total peripheral vascular resistance (TPR).
BP = CO × TPR
Cardiac output is the amount of blood that passes a point within a blood vessel per unit of time and is most often displayed as liters per minute. The three main factors that determine cardiac output are preload, afterload andcontractility. The autonomic nervous system also contributes extensively to cardiac output through its regulatory effects on vascular tone, inotropy and chronotropy of the heart.
Total peripheral resistance is the impediment to blood flow in a vessel, and approximately 2/3 of vascular resistance is in the small, muscular arterioles. Resistance cannot be measured directly; instead it is calculated from measurements of blood flow and pressure difference.
Poiseuille’s law helps to explain conductance through blood vessels, where conductance is a measure of the blood flow through a vessel for a given pressure difference.
Q=(πΔPr4)/8ηl
Q= blood flow; ΔP = pressure difference between the two ends of the vessel; r = radiusof the vessel; η = blood viscosity, l = length of the vessel.
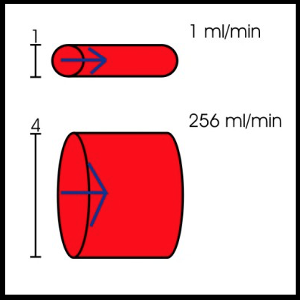
It is most important to recognize that flow through a vessel will be directly proportional to the pressure difference within the vessel and will increase to the fourth power of the radius of the vessel. Flow will be indirectly proportional to the viscosity of the blood and to the length of the blood vessel.
Tight regulation of arterial blood pressure is important for a number of reasons. Delivery of the proper amount of blood to tissues is vital for the delivery of oxygen and nutrients, removal of carbon dioxide and waste products, for maintenance of ionic concentrations required for transmembrane potentials and depolarization, and for transport of humoral substances, i.e., hormones. It has been documented that an eight-fold increase in metabolism results in an approximately four-fold increase in blood flow to the active tissues, while a decrease in arterial oxygen saturation from 100% to 25% will result in a three-fold increase in blood flow. Blood pressure regulation has both acute control mechanisms and long- term control mechanisms.
The acute control mechanism is most readily explained by the vasodilator theory. It is proposed that with decreases in available oxygen and nutrients, cells produce an increased amount of an unknown vasodilator substance (likely nitric oxide) that diffuses back to the precapillary sphincters, metarterioles, and arterioles, thereby resulting in vasodilation. The increase in blood flow will wash out the vasodilator substance and decrease its production after re- establishment of proper oxygen concentrations. Another vasodilation control theory is the oxygen demand theory. It is hypothesized that oxygen and nutrients are required to maintain smooth muscle contraction, and in the absence of these required substances, arteriolar vasodilation would occur. The problem with this theory is that smooth muscle contraction has been identified to persist for long periods of time in the presence of extremely low concentrations of oxygen-even below those found normally in tissues.
Special mechanisms also exist in some tissues to auto-regulate local blood flow. The juxtaglomerular apparatus within the kidneys serves to detect fluid composition in the early distal tubule. In the face of hyponatremia or hypovolemia, the afferent renal arteriole vasodilates in an effort to increase glomerular filtration rate and the efferent renal arteriole vasoconstricts to increase the glomerular capillary pressure. The cerebral blood vessels will undergo vasodilation not only in response to decreases in the amount of available oxygen but will also vasodilate in response to increases in the carbon dioxide or hydrogen ion concentration. The increased blood flow serves to washout the increased concentrations of carbon dioxide or hydrogen ions. It is believed the carbon dioxidecombines with water to form carbonic acid. The carbonic acid dissociates to form hydrogen ions that result in vasodilation of the cerebral blood vessels. The fact that hydrogen ions result in vasodilation is important as a protective response given that neuronal activity is greatly depressed in the face of acidosis.
The long-term blood pressure control yields more complete regulation than the acute mechanisms and serves to change as the metabolic demands of the tissues change. The most common mechanism for long-term control is a change in the degree of vascularity of the tissues. It is believed that oxygen is a primary determinant of angiogenesis where animals exposed to lower oxygen concentrations display increased vascularity. Angiogenic factors are numerous, i.e., endothelial cell growth factor, fibroblast growth factor, angiogenin, and are most commonly released from 1) ischemic tissues, 2) tissues displaying rapid growth, and 3) tissues displayingexcessively high metabolic rates. These angiogenic factors cause new vessels to sprout from small venules or capillaries, with infiltration of smooth muscle cells into the new vessels, thus yielding small arterioles.
There are also a large number of humoral factors that result in vasoconstriction or vasodilation of the arterioles, resulting in increased or decreased total peripheral vascular resistance, respectively. The most potent vasoconstrictors recognized include endothelin, angiotensin II, norepinephrine and antidiuretic hormone (vasopressin). Vasodilators include nitric oxide, adenosine, serotonin, bradykinin, histamine, and some prostaglandins.
The two most common diseases recognized with systemic hypertension in dogs are renal disease and hyperadrenocorticism. Nephrosclerosis and hypoxic nephrons result in activation of the renin-angiotensin system with eventual production of angiotensin II and aldosterone. The diseased kidney may not excrete salt and water properly, resulting in fluid retention, increased cardiac output and total peripheral resistance. Mechanisms contributing to hypertension with Cushing’s syndrome include excessive secretion of renin, activation of the renin-angiotensin system via alternative stimulators, enhanced vascular sensitivity to pressors, reduction of vasodilator prostaglandins, and increased secretion of mineralocorticoids. Essential hypertension (idiopathic) has been reported along with hyperaldosteronism, pheochromocytoma, and polycythemia as causes for elevated blood pressure.
The two most common diseases recognized in cats with systemic hypertension are renal disease and hyperthyroidism. Despite a decrease in the total peripheral resistance, many hyperthyroid cats are hypertensive as a consequence of increased cardiac output and direct sympathomimetic effects on the vasculature. Renaldisease results in hypertension via similar mechanisms encountered in the dog.
Clinical signs of systemic hypertension vary with the target organ that is involved. The most frequent target organs are the eyes, kidneys, cerebrovascular and cardiovascular systems. Severe systemic hypertension frequently results in acute blindness secondary to retinal hemorrhage, retinal detachment, and/or hyphema. The renal changes associated with hypertension may be secondary sclerotic changes, or frequently, may be the primary disease entity with secondary hypertension. Regardless of which comes first, animals with renal disease and hypertension often display polyuria/polydipsia (due to pressure diuresis or primary renal disease) andproteinuria. Glomerular atrophy and nephron loss are often seen along with tortuous intrarenal vasculature.Multifocal hemorrhage and associated neurologic signs may occur secondary to intracranial bleeding or infarcts,and central nervous system arteriosclerosis and vasospasm have been recognized. The cardiovascular response to systemic hypertension involves concentric hypertrophy of the left ventricle. Heart failure occurs infrequently in animals with only hypertension, but in the face of other cardiovascular diseases, the combination may result in congestive signs (i.e., pulmonary edema, ascites, pleural effusion).
ECG changes associated with left ventricular hypertrophy will include increased R wave amplitude and QRSduration. Thoracic radiographs are often unremarkable in dogs because the concentric hypertrophy of the left ventricle does not change the cardiac silhouette (increase in wall thickness rather than the radius of the chamber).Cats frequently display an enlarged aortic root and an elongated/serpentine aorta associated with hypertension as the vessel loses elasticity. Many animals with systemic hypertension will have soft systolic murmurs over the sternum or left heart base as a consequence of turbulent blood flow within the right ventricular outflow tract. It is believed this tract is narrowed because of impingement from the concentrically hypertrophied interventricular septum. Echocardiographically, we commonly recognize the left ventricular concentric hypertrophy and right ventricular outflow tract obstruction.
References
- Bach JF, Rozanski EA, MacGregor J, Betkowski JM, Rush JE. Retrospective evaluation of sildenafil citrate as a therapy for pulmonary hypertension in dogs. J Vet Int Med 2006;20:1132-35.
- Campbell FE. Cardiac effects of pulmonary disease. Vet Clin Small Anim 2007;37:949-62.
- Johnson L, Boon J, Orton EC. Clinical characteristics of 53 dogs with Doppler-derived evidence of pulmonary hypertension: 1992 – 96. J Vet Int Med 1999;13:440-7.
- Kellum HB, Stepien RL. Sildenafil Citrate Therapy in 22 Dogs with Pulmonary Hypertension. J Vet Int Med 2007;21:1258-64.
- Pyle RL, Abbott J, MacLean H. Pulmonary hypertension and cardiovascular sequelae in 54 dogs. Intern J Appl Res Vet Med 2004:2:99-109.
- Zabka TS, Campbell FE, Wilson DW. Pulmonary Arteriopathy and Idiopathic Pulmonary Arterial Hypertension in Six Dogs. Vet Pathol 2006;43:510-22.
- Littman, M.P., Fox, P.R., Systemic Hypertension: Recognition and Treatment, In: Fox PR,
- Sisson DD, Moise NS, editors. Textbook of Canine and Feline Cardiology: Principles and Clinical Practice, 2nd ed. Philadelphia: WB Saunders; 1999. p. 795-813.