Overview
Broadly speaking, I focus on extragalactic astronomy. Specifically, I focus on leveraging various dynamical systems and large astronomical data sets to research the nature of dark matter/energy and galaxy formation and evolution. Below is a bit about some projects I’m currently working on, as well as a little info on some topics I’m just generally interested in!
The High-Redshift Universe
JWST has already revolutionized our understanding of the early universe, and continues to do so every week. For example, how and when did the first galaxies form? How fast were they able to build up and evolve their stellar populations? I am privileged to be working working with the CANUCS (The CAnadian NIRISS Unbiased Cluster Survey – JWST Cycle 1 GTO Program) collaboration, combined with the “Technicolor” (Cycle 2 GO) data to derive properties of some of the first galaxies (6<z<12), and test them against our current models of galaxy formation and evolution.
HSC-Joint “u2-CH2” catalog
As a part of the Subaru Prime Focus Spectrograph (PFS) collaboration, I worked to include the reddest bands, de-blended Spitzer IRAC (CH1 and CH2) photometry to the “u2k” catalog. Covering ~18 square degrees of IRAC footprint in the EN1, DEEP-23, COMSOS and XMM-LSS fields. The inclusion of these reddest bands are critical to constrain the properties of higher redshift sources, such as stellar mass and redshift. These fields are also targets for the upcoming PFS survey, and combined with the”u2-CH2″ catalog will provide an unprecedented combination of excellent spectroscopy and photometry from the ultraviolet through to 4.5 microns. I am very excited for the incredible breadth of science that will be possible due to this combination of the incredible PFS capability combined with the photometric catalogs we have created.
Near-field Cosmology
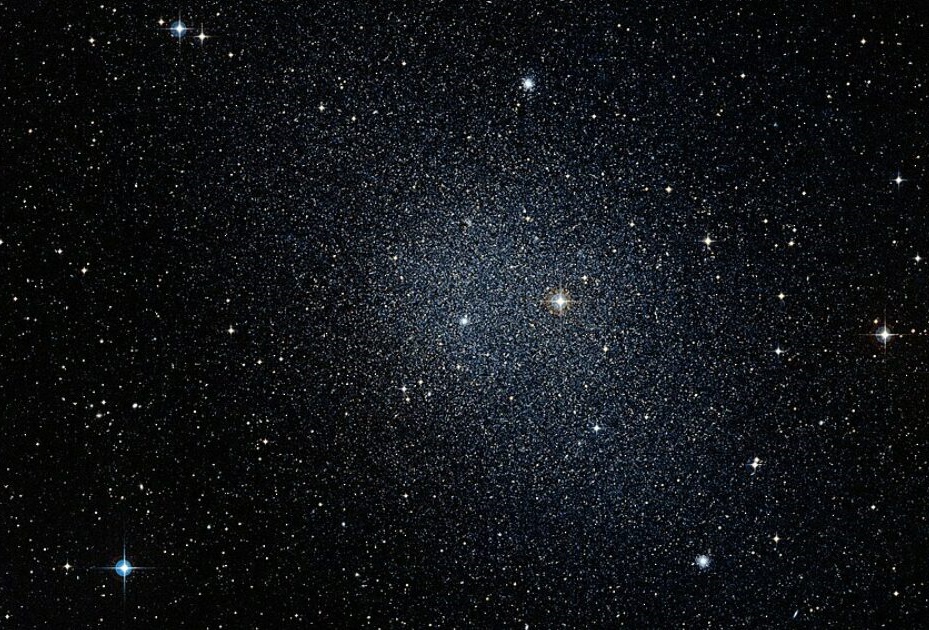
Many of the central discrepancies in today’s modern LCDM cosmological framework arise at small (galaxy-system) scales. On large scales, LCDM has enjoyed a lot of success modeling how the linear growth of small perturbations in the density field in the early universe (which we observe in the Cosmic Microwave Background, or CMB), grew into the vast diversity of structure we observe at today, at z = 0. However, on smaller scales — i.e. on galaxy-system scales (for example, the Milky Way – Andromeda system), LCDM faces a few critical challenges, such as the so-called ‘core-cusp’ problem.
Therefore, studying the formation histories, kinematics, and interaction histories of these small-scale galaxy systems, such as ultra-faint dwarf galaxies, provides critical tests of LCDM, as well as other cosmological models.
Galaxy Clustering/Power Spectra
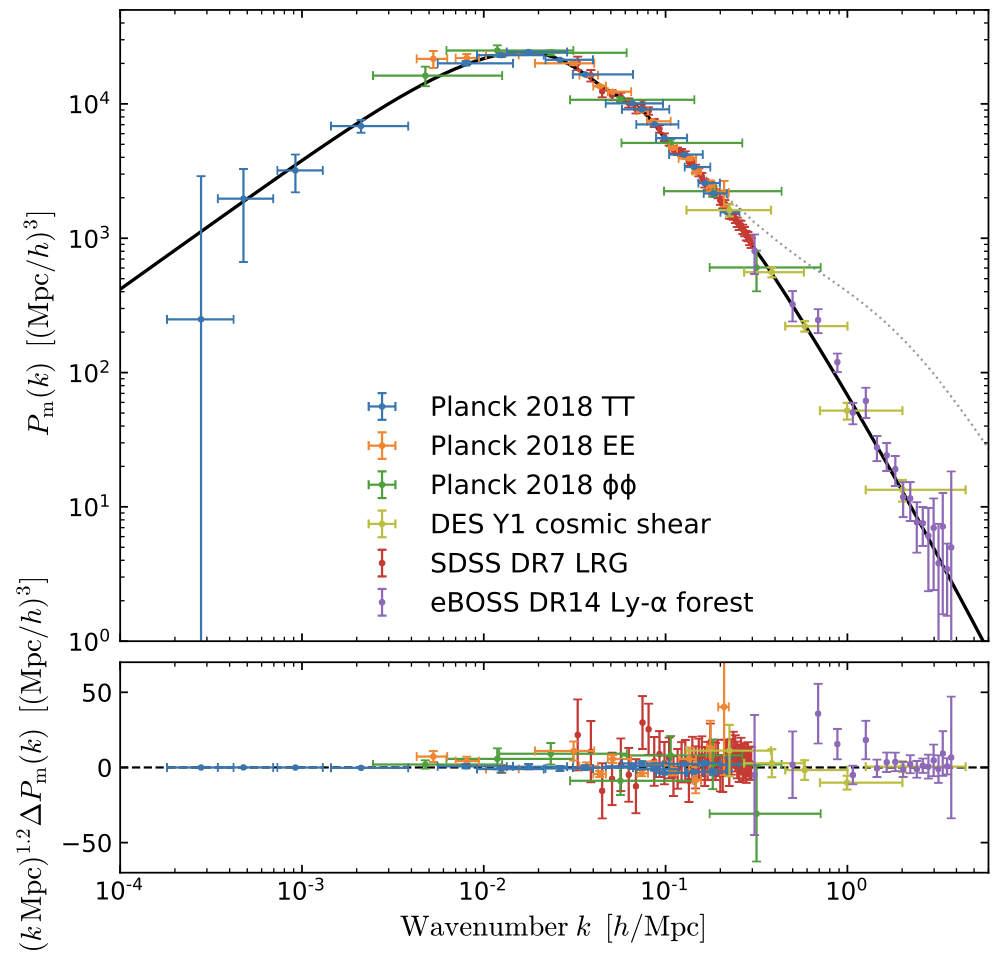
If you count the number of pairs of galaxies that are a certain distance apart, and then repeat the count for a range of separations, you build what is known as a two-point correlation function. Then, if you take the Fourier transform of that correlation function, you get the galaxy power spectrum. Next, if you include galaxy clusters, the cosmic microwave background and various objects measured in the Lyman-alpha forest in your counting, then you build the matter power spectrum of the universe. This power spectrum in very sensitive to the cosmology that you’re using, and therefore is a very powerful tool that can be used to constrain the nature of dark matter, dark energy and models of the growth of large-scale structure in our universe.
Galaxy Rotation Curves
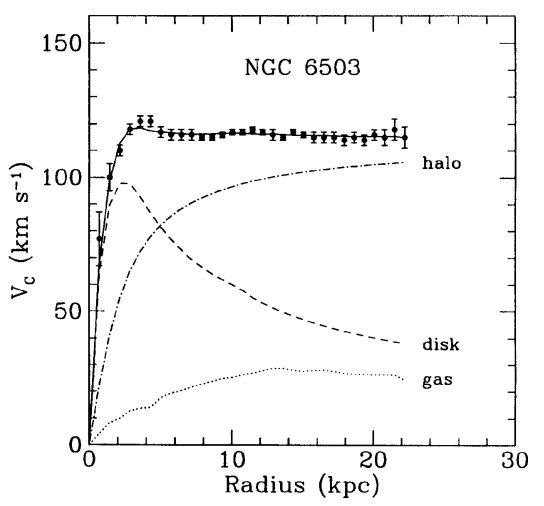
In the 1970’s, Vera Rubin was the first to observe that the kinematics of disk-like galaxies had large discrepancies from how these galaxies should spin based only off of how much matter we could actually see. Since then, disk galaxies have remained one of the most important pillars of evidence of the missing mass problem (which is solved by the introduction of Dark Matter, or modifying our models of gravity). The difference between the predicted (baryonic) rotation curve and the actual observed rotation curve is, in my opinion, most concisely represented by the Radial Acceleration Relation (RAR) in late type galaxies (below).
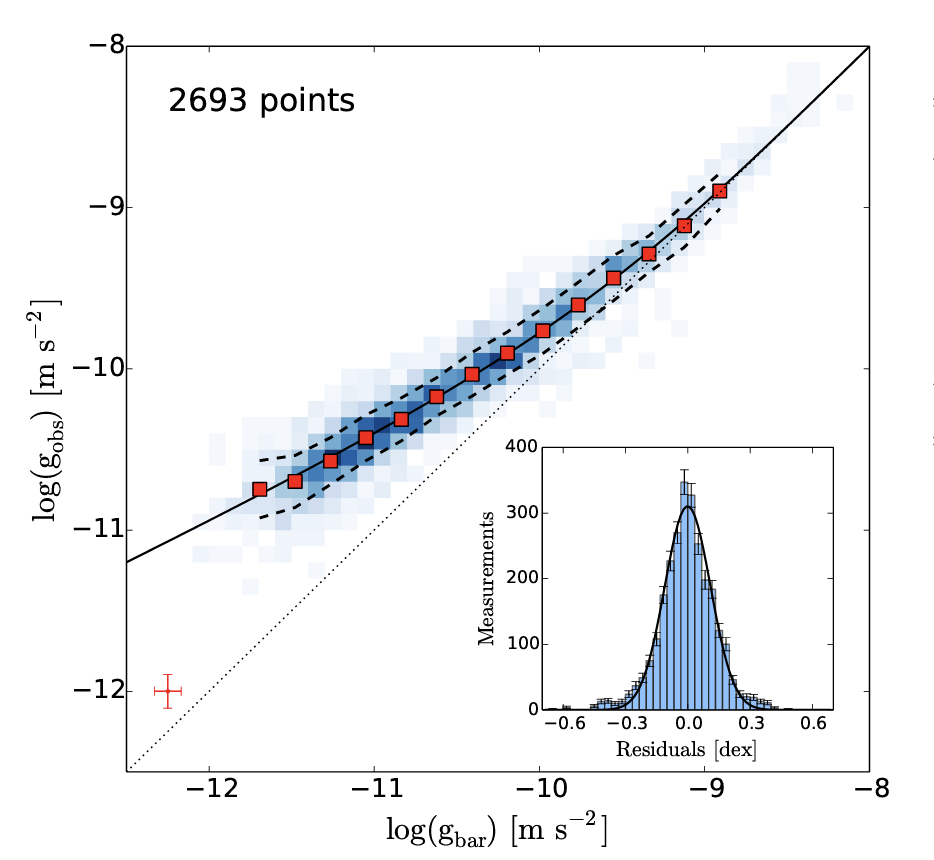
The RAR is built by determining the baryonic and observed circular radial acceleration at different radii for 175 late type galaxies, and then over-plotting each data point in a log-log scale. At the high acceleration range, the RAR falls on the 1:1 line, indicating that all the acceleration present in the central regions of disk galaxies can be accounted for by the baryons alone. However, at the low acceleration range the observed acceleration is up to an order-of-magnitude higher than what can be caused by only the baryons, indicating that at large radii disk galaxies require the exertion of some extra force to explain their accelerations. This extra force can be modeled either by the introduction of non-baryonic dark matter, or by modifying how gravity behaves below the critical acceleration scale of a0 = 1.2 angstroms per second squared.
To see for yourself how dark matter changes the rotation curves of LTG’s, try the Spiral Galaxy Rotation Curve Builder, made by Prof. David Wittman at UC Davis.