We study the biomechanics and neural control of swimming in fishes. In any animal, locomotion requires a complex set of nested feedback loops. For example, the brain activates a neural circuit called a central pattern generator (CPG) that produces the basic pattern of muscle activity for swimming in fish, for flying in insects, and for walking in humans. The CPG activates muscles that produce force to bend the body, which interacts with the fluid around it. But the fluid also produces forces back on the body, and the body movement affects how much force the muscle can produce. The CPG then responds to sensory inputs, changing the pattern of muscle activity to respond to perturbations, such as turbulence in the water for fishes, or uneven ground for walking animals. The brain receives further sensory inputs from senses such as vision, the vestibular system, and the lateral line, an extra sense that fish have to detect water moving around, which then allow it to modulate the CPG in more complex ways. These circuits in individual animals interact with those in others, leading to complex social behavior like schooling.
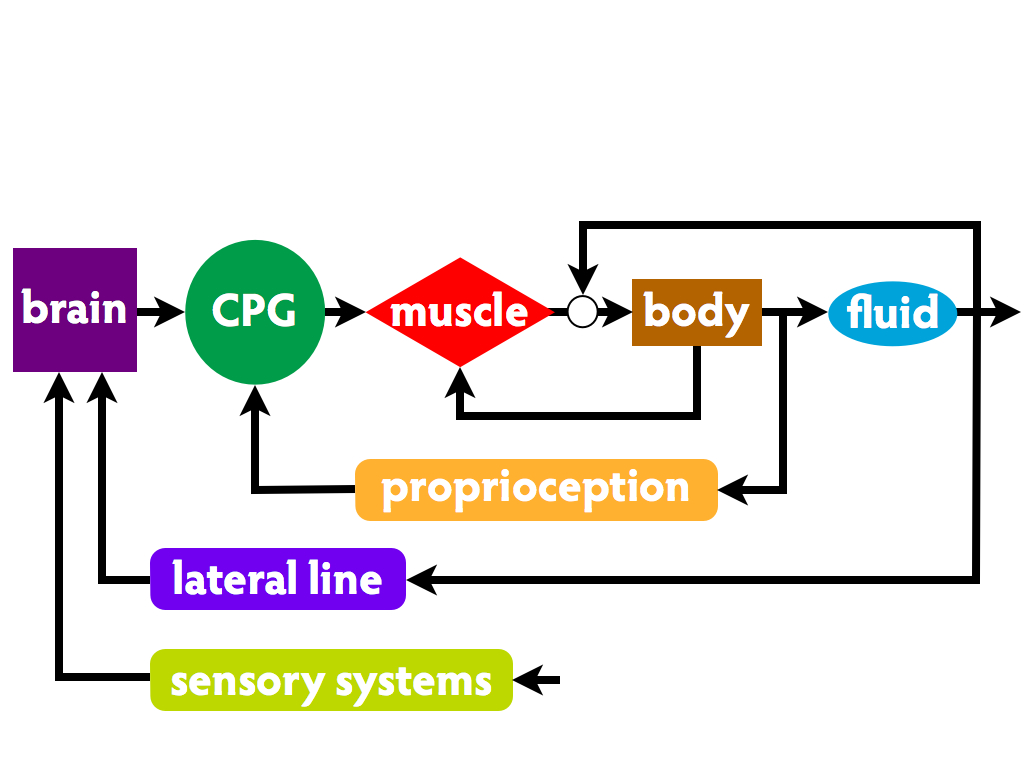
Work in the laboratory focuses on the interaction of all these different components, using engineering techniques to study water moving around fish, as well as biomechanical and neurophysiological techniques to examine sensory systems and neural control systems.
Fluid dynamics
We use a technique called particle imaging velocimetry (PIV) to visualize how water moves around fish as they swim. A high power laser is used to illuminate a single plane in the tank where the fish is swimming.
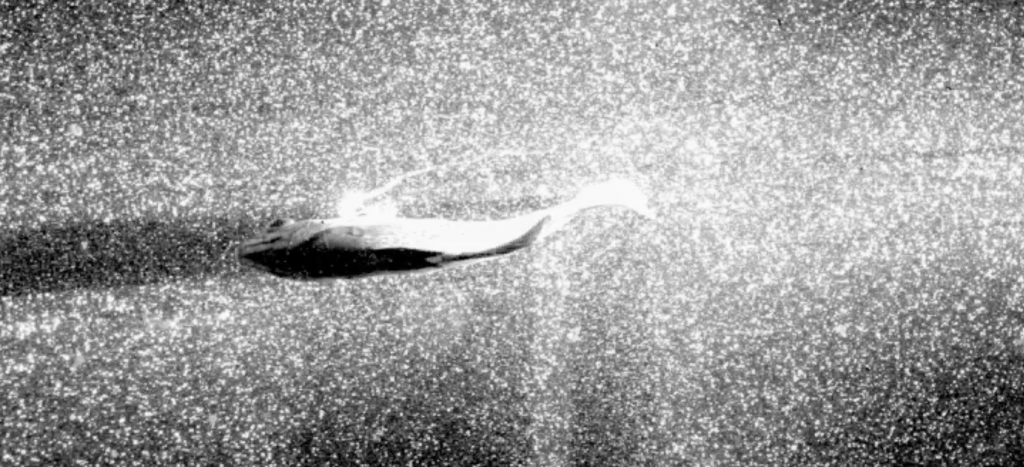
Small reflective particles are placed in the water, and a camera is used to track how those particles move in the water around the fish. This lets us understand better the forces that a fish must deal with as it swims through turbulent water, and also the forces that a fish produces using its fins in order to switch effectively, turn rapidly, and maintain stability. We are particularly interested in understanding the fish’s body and its fins, which are flexible, bend in response to both internal muscular forces and external fluid forces. This fluid-structure interaction may actually allow the fish to respond more effectively and more rapidly to external perturbations, such as turbulent eddies.
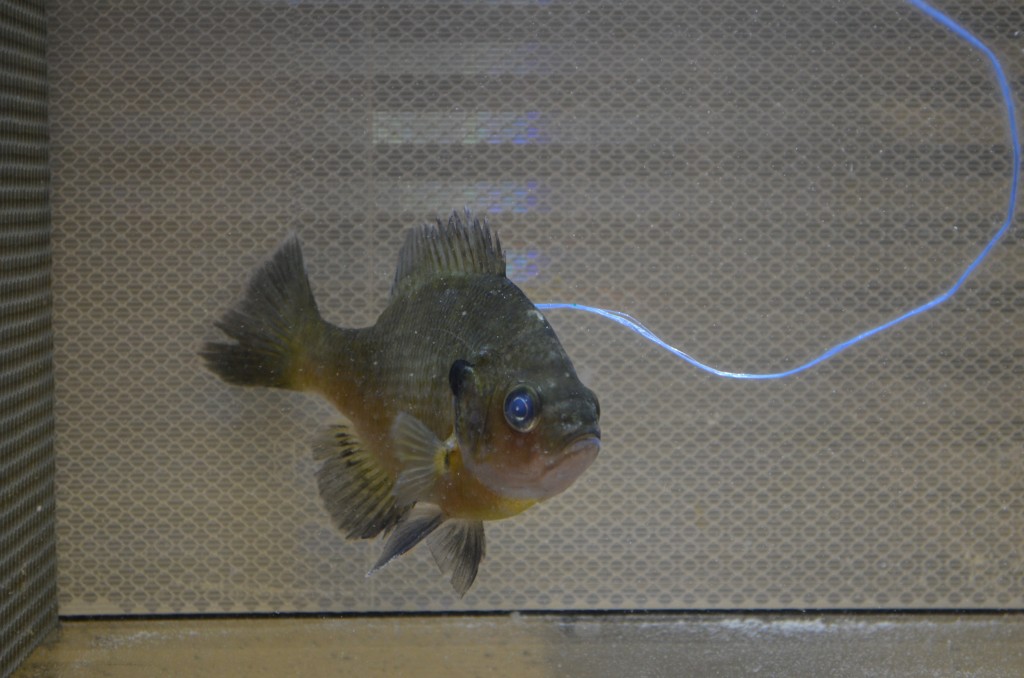
To address these questions, we also use computational simulations in collaboration with other laboratories. These simulations allow us to make “what if” experiments that would not be possible in reality. For example, what if a fish’s body was twice as stiff? Or, what if its muscles were twice as strong?
Body mechanics and muscle physiology
How stiff is a fish’s body? Most fish bodies are quite flexible, and can bend under the forces from the fluids around them. But they can also vary their effective body stiffness by activating muscle. We are studying how these muscular changes in body stiffness contribute to swimming performance, and how the intrinsic properties of muscles can help animals respond to perturbations in their environment. Muscle is a very complex, active material. When an animal is hit by an unexpected perturbation, like a vortex in the water, the muscle begins to change how much force it produces – even before any sensory system can detect the perturbation. We use isolated muscle preparations and whole-body preparations to measure how muscle can respond to perturbations, even as it produces energy for locomotion.
Schooling behavior and sensory physiology
We study how sensory systems, neural circuits, and biomechanics come together to allow fish to form and maintain schools. For this behavior, they need to be able to reliably sense their neighbors, even when sensory information is limited or degraded. We use tests on small schools of fish under different sensory conditions to probe how fish sense each other.
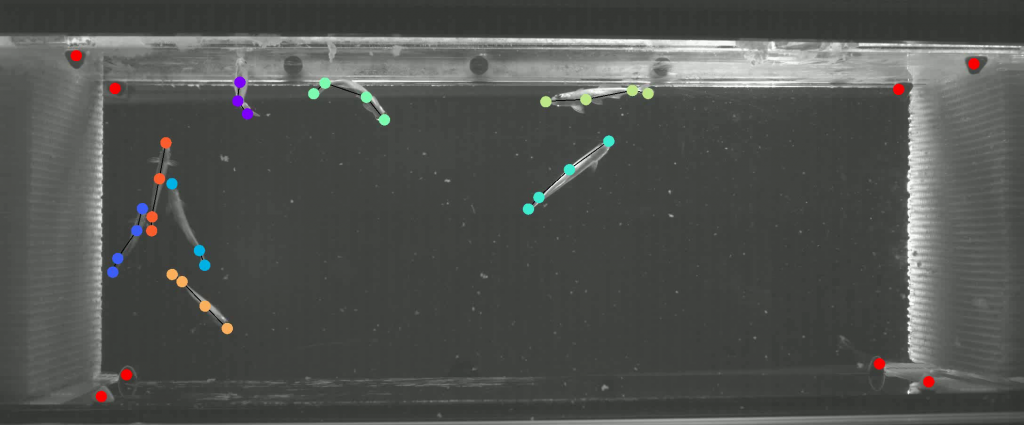