Research Videos
Videos of some conference talks.
Active Research Summaries
Updated September 19, 2024.
Ultrasonic Anemometer for Wind Measurement on Mars
Collaboration with Don Banfield at NASA Ames and Ian Neeson at VN Instruments, Ontario
Students: Kevin Ligonde, Xinyu Shi, Spencer Schmid, Tim Cheng, Julia Huckaby, Tara Curran, Friedlay Steve, Rishabh Chaudhary, Ben Fisher, Zarina Kosherbayeva
A sonic anemometer is under development for use in low pressure, low temperature environments such as the surface of Mars or the upper atmosphere of the Earth. This technique allows simultaneous measurement of wind speed and the speed of sound by measuring the acoustic time of flight over multiple acoustic transmission paths. Using 3 non-planar paths, three-dimensional information on flow speed can be recovered. At 6 mbar in CO2 (conditions at the Martina surface), we have achieved resolutions of better than 5 cm/s at flow speeds below 0.5 m/s, accuracy of better than 3% at flow speeds up to and above 12 m/s. Update rates of 20 Hz should be achievable. The primary challenge of operating an acoustic anemometer on Mars is production of high enough signal to noise ratios in the thin (~ 6-11 mbar) CO2 atmosphere. A second concern is the low temperature of operation and the impact that temperature changes may have on acoustic transducer performance. We are able to demonstrate flow measurement at pressures as low as 2 mbar. There are multiple versions of the Tufts Sonic (built at Tufts) and the Mars Sonic (built at VN Instruments and NASA Ames Research Center).
Versions of the Tufts Sonic have been tested at the University of Aarhus Mars Simulation Wind Tunnel (2019 and 2023), and have flown on 4 stratospheric balloon missions to date (Fort Sumner, New Mexico) in 2021, 2022, 2023 and 2024 to altitudes as high as 38 km (130,000 feet). Two additional flights are planned out of Antarctica and New Zealand in 2024/2025. Glad to be working with Don Banfield and NASA Ames Research Center, Chris Yoder at NASA Wallops Balloon Programs Office, and Ian Neeson at VN Instruments.
![]() | ||
![]() |
![]() |
![]() |
Representative Publications:
Robert D. White, Rishabh Chaudhary, Zijia Zhao, Luisa Chiesa, Ian Neeson, and Don Banfield “Modeling and characterization of gas coupled ultrasonic transducers at low pressures and temperatures and implications for sonic anemometry on Mars” Journal of the Acoustical Society of America 156, pp 968-988, (2024). LINK
Across Acoustics, “Ultrasound Transducers for Measuring Martian Wind Speeds” ASA Publications Office Podcast, Robert White and Kat Setzer, August 26, 2024. https://www.buzzsprout.com/1537384/15607299
Robert D. White, Rishabh Chaudhary, Tim J. Cheng, Benjamin Fisher, Julia Huckaby, Donald Banfield, Anthony Colaprete, Amanda Cook, Arwen Dave, Vandana Jha, Abraham Rademacher, Jared Shimada, Bruce White, Christopher Yoder, Ian Neeson, Jonathan Merrison and Jens Jacob Iversen. “Flow Testing of a Digital Sonic Anemometer for Martian and Stratospheric Environments,” AIAA 2024-3933. AIAA AVIATION FORUM AND ASCEND 2024, July 2024. LINK
R. D. White, I. Neeson, E. S. Schmid, J. Merrison, J. J. Iversen, and D. Banfield, “Flow Testing of a Sonic Anemometer for the Martian Environment,” in AIAA Scitech 2020 Forum, Orlando, Jan 6-10 2020. AIAA2020-0712 LINK
AIP Press, “Measuring Martian Winds with Sound“, August 13, 2024. https://publishing.aip.org/publications/latest-content/measuring-martian-winds-with-sound/
Stratospheric Sonic Anemometer
Collaboration with Don Banfield, Chris Yoder (NASA) and Ian Neeson (VN Instruments). Students: Tim Cheng, Julia Huckaby, Tara Curran, Friedlay Steve, Rishabh Chaudhary, Ben Fisher, Zarina Kosherbayeva
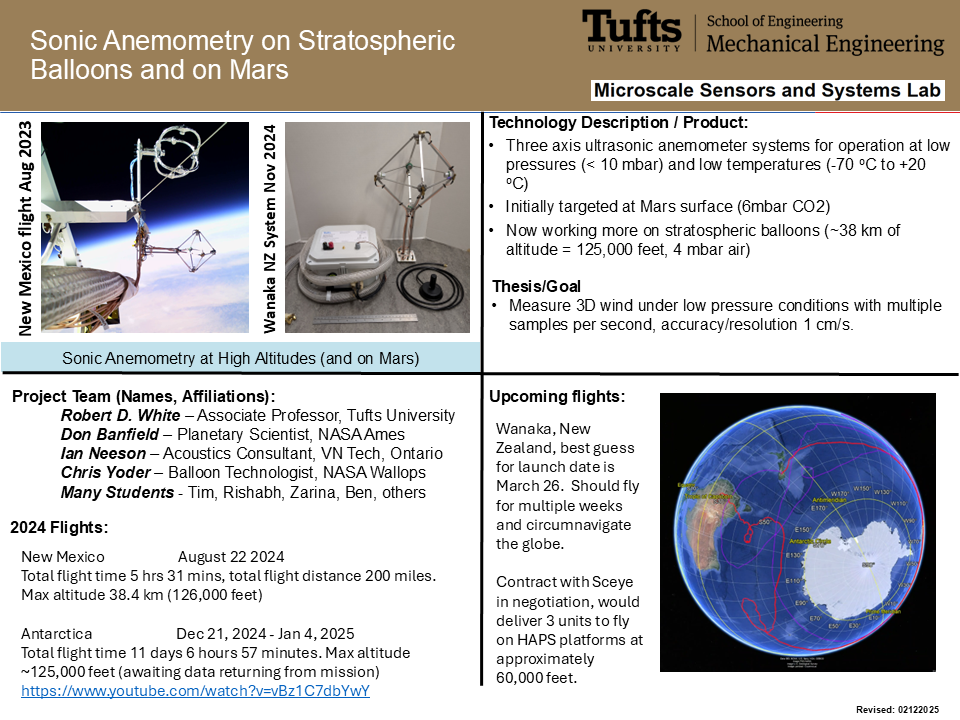
Robert D. White, Rishabh Chaudhary, Tim J. Cheng, Benjamin Fisher, Julia Huckaby, Donald Banfield, Anthony Colaprete, Amanda Cook, Arwen Dave, Vandana Jha, Abraham Rademacher, Jared Shimada, Bruce White, Christopher Yoder, Ian Neeson, Jonathan Merrison and Jens Jacob Iversen. “Flow Testing of a Digital Sonic Anemometer for Martian and Stratospheric Environments,” AIAA 2024-3933. AIAA AVIATION FORUM AND ASCEND 2024, July 2024. LINK
Across Acoustics, “Ultrasound Transducers for Measuring Martian Wind Speeds” ASA Publications Office Podcast, Robert White and Kat Setzer, August 26, 2024. https://www.buzzsprout.com/1537384/15607299
Tim J. Cheng, Chris Yoder, Tara Curran, Freidlay Steve, Don Banfield, and Robert D. White, “Test Flight of a Stratospheric Sonic Anemometer Prototype” Journal of Atmospheric and Ocean Technology, published online October 17, 2024. https://doi.org/10.1175/JTECH-D-24-0010.1
Ultrasonic Gas Composition for Planetary Science
Collaboration with Don Banfield at NASA Ames, Andi petculescu at Univ of louisiana
Students: Rishabh Chaudhary
We are working with collaborators at NASA and the Univ of Louisiana to build an ultrasonic system for measuring gas composition using ultrasonics. The concept is targeted at measuring the H2:He content and H2 Ortho:Para ratio in the atmospheres of the giant planets (e.g. Saturn) on a descent probe. Computational and laboratory work is ongoing to validate the concept and produce a functional prototype.
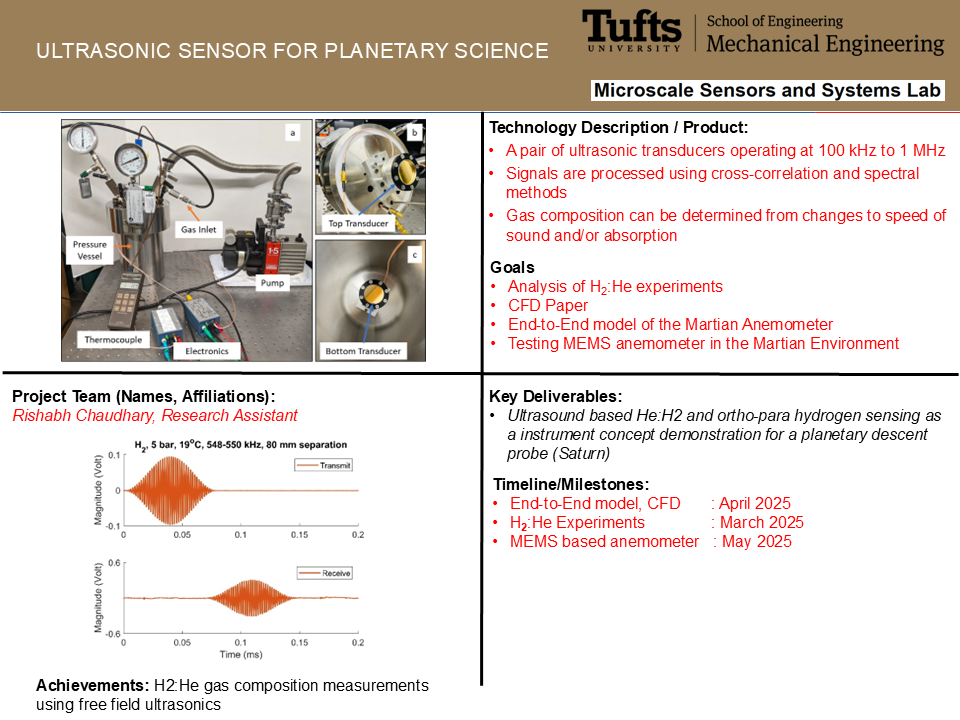
Representative Publications:
Rishabh Chaudhary, Robert D. White, Zarina Kosherbayeva, Donald Banfield, Anthony Colaprete, Ian Neeson, Andrew Powell and Andi G. Petculescu. “Ultrasonic Measurement of Helium Abundance in Hydrogen for Planetary Science,” AIAA 2024-3851. AIAA AVIATION FORUM AND ASCEND 2024, July 2024. LINK
Jha, Vandana, Colaprete, Anthony, Banfield, Donald J. , Cook, Amanda M. , White, Bruce , Dave, Arwen I, Agrawal, Parul , Rademacher, Abraham , Shimada, Jared A. , White, Robert D. , Chaudhary, Rishabh , Neeson , Ian, Powell, Andrew, Petculescu, Andi, Rustemeyer, Nancy L. “Atmospheric Structure Investigation: Planetary In Situ Measurements of Atmospheric State, Including Clouds/Aerosols, Hydrogen Ortho/Para Fraction, and Helium Abundance on Saturn or Uranus” in the American Geophysical Union Fall Meeting, Washington, DC Dec 9-13, 2024. (AGU24)
MEMS Hydrophone
Students: souleymane Cissokho
In collaboration with MIT Lincoln Labs and industry partners SeaLandAire Technologies and Navmar Applied Sciences Corporation, we are developing a MEMS hydrophone based around a commercial MEMS microphone part. This sensor allows smaller size and lower cost than existing Navy hydrophones, but with a comparable or improved performance. Project includes computational modeling, system electronics design and fabrication, prototype unit manufacturing, and calibrator and pool testing.
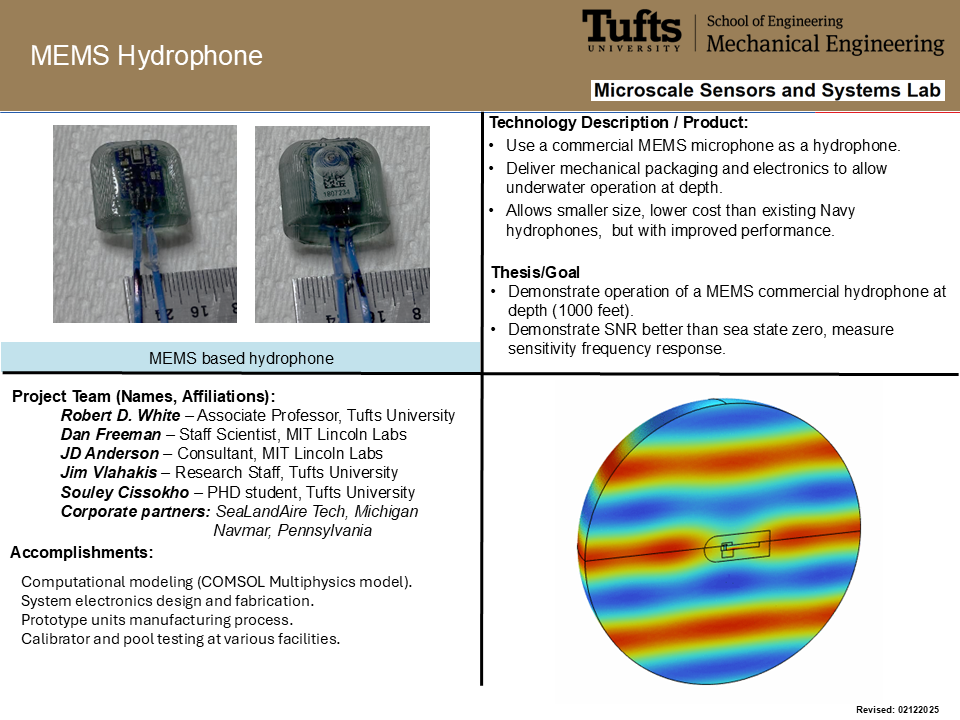
Aluminum Nitride Thin Films
Students: maijie xiang, cade smith, mischael anilus, freidlay steve
In order to fabricate our own MEMS microphones, ultrasound transducers and pressure sensors, we need the ability to deposit, pattern, and characterize piezoelectric thin films. In this project we are developing our capabilities to work with Aluminum Nitride thin films for MEMS sensor applications. This is not novel, others have done this, however we need to develop our capabilities in this area either with our own deposition chamber or working with vendors or collaborators. This activity is at the process development and materials testing stage but will lead to device design.
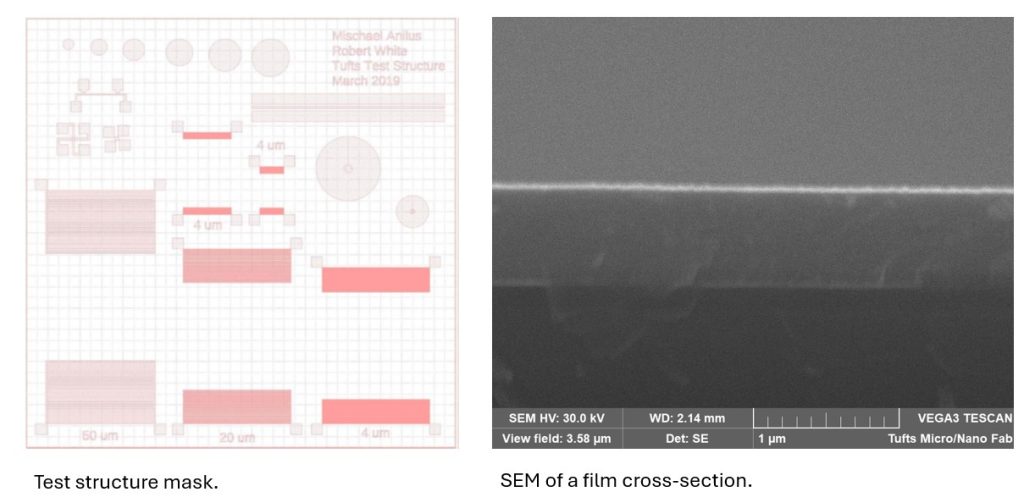
Representative Publications:
Freidlay Steve, Robert Littrell, Craig Core, Don Banfield, and Robert D. White, “Aluminum Nitride Piezoelectric Micromachined Ultrasound Transducers with Applications in Sonic Anemometry”, in the 181st meeting of the Acoustical Society of America, Seattle, WA, Nov 29 – Dec 3 2021. https://pubs.aip.org/asa/jasa/article-abstract/150/4_Supplement/A96/705970
Infrasound Measurement
Students: Cade Smith
Infrasound measurement on stratospheric balloons for atmospheric gravity wave detection and energetic event detection.
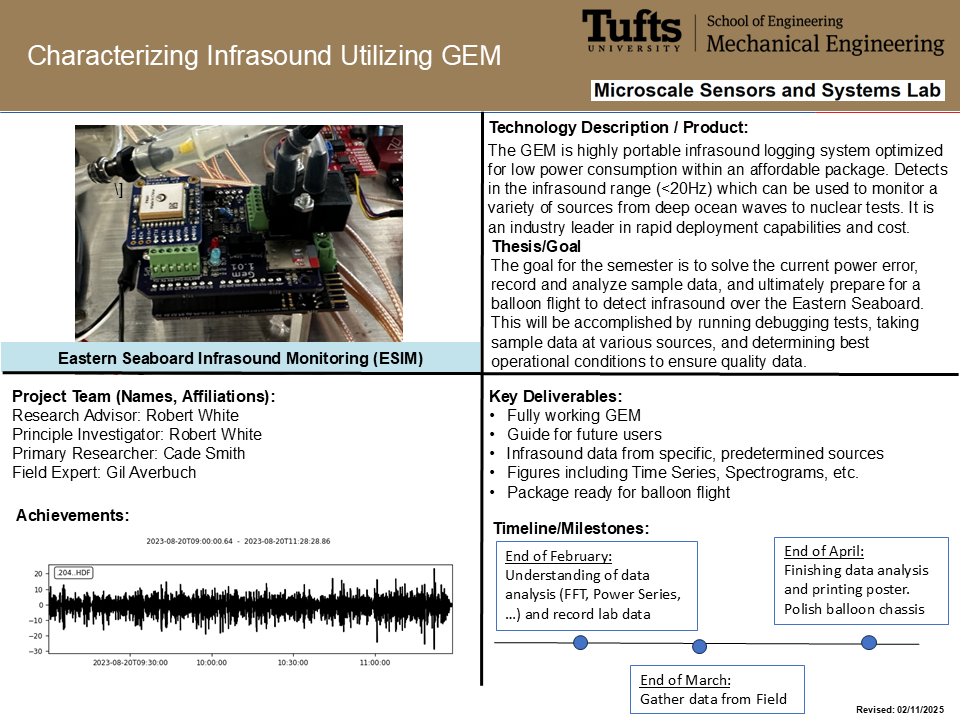
Wind Tunnel Construction
Students: ethan laverack, yucheng shi, steven rauso, owen swint, Sean doherty, andy navarro, owen swint
The lab is in the process of building a high speed, small cross-section wind tunnel for sensor testing. The design is a recirculating tunnel targeting a Mach number of 0.5 in the test section. Upstream screens and honeycombs attempt to reduce turbulence. Many students have contributed to design and construction, hopefully the tunnel will be online soon!
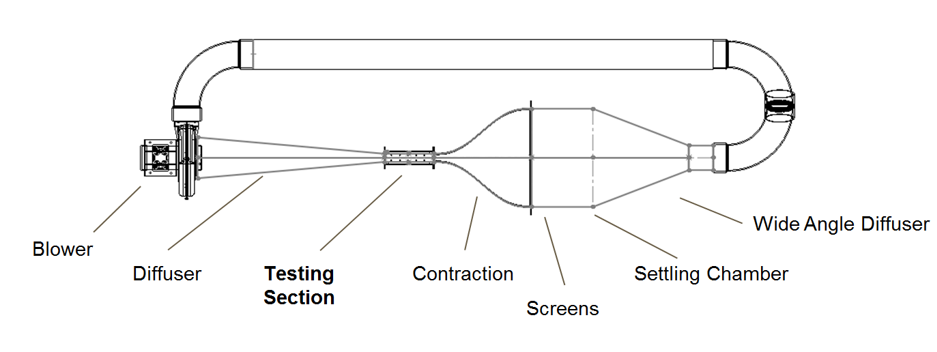
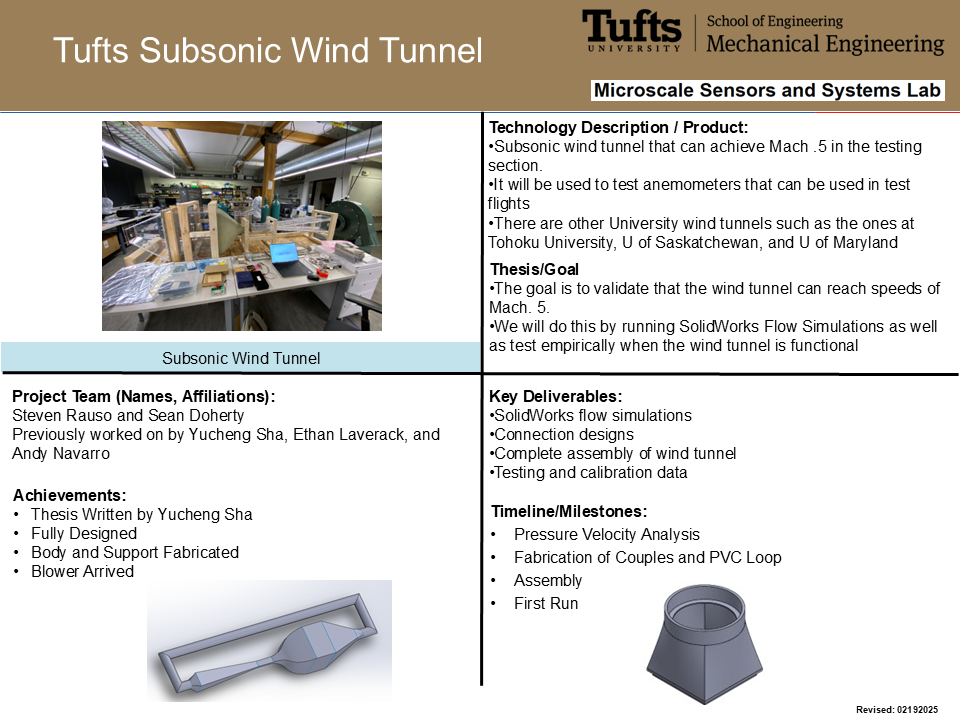
Vibrotactile Display
With Jon Bernstein. Students: Maijie Xiang
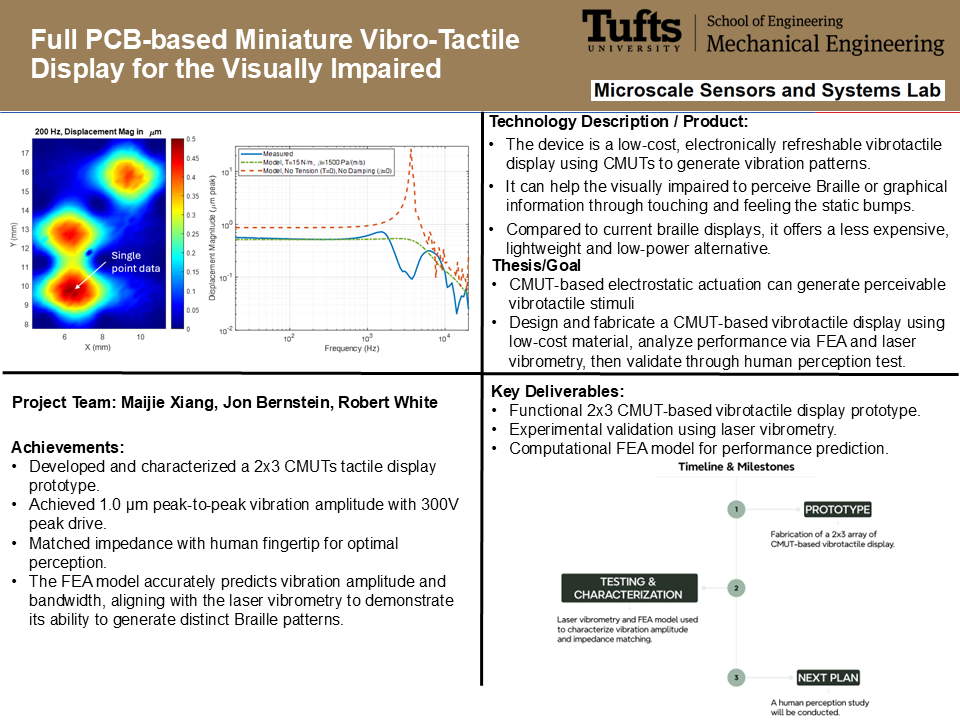
Maijie Xiang, Robert D. White, Jonathan Bernstein , “PCB-based Miniature Vibro-Tactile Display for the Visually Impaired” in the 186th Meeting of the Acoustical Society of America, Ottawa, May 13-17, 2024.
MEMS Concertina Springs
Student: Tim Cheng, Draper Scholar
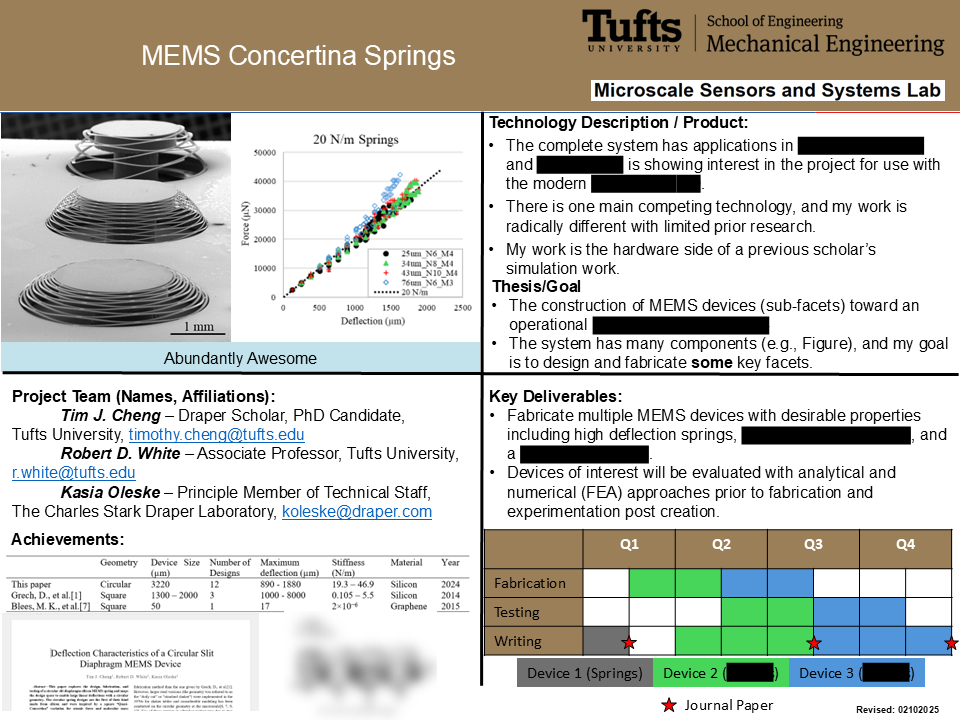
Miniaturized Ultrasonic Anemometer
Students: Jean Pascal Cyusa Shyaka, Rishabh Chaudhary
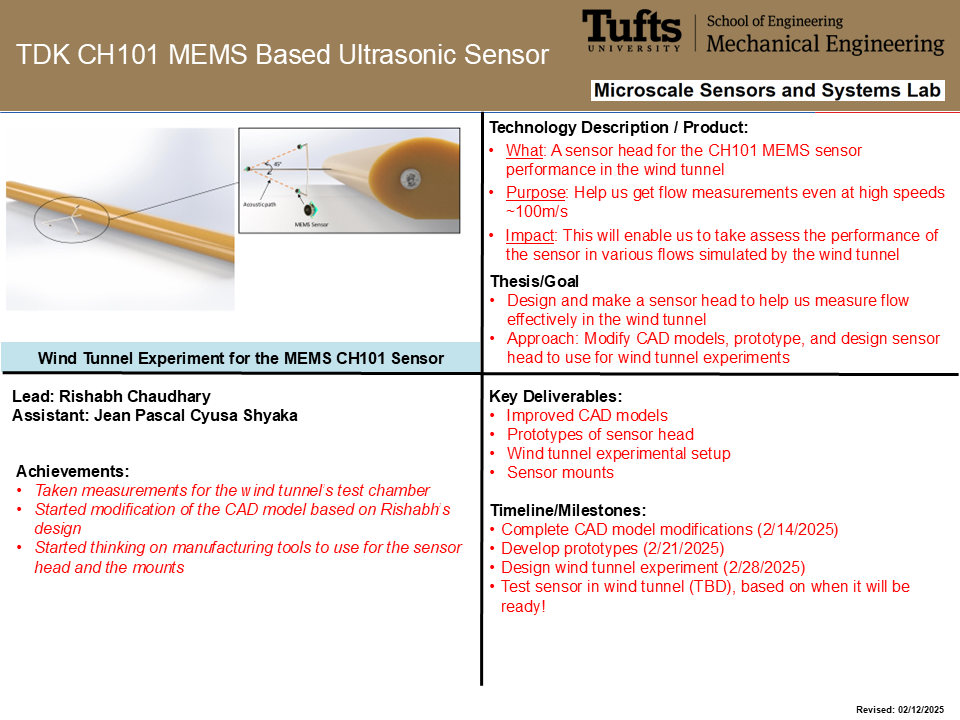