Projects Overview
- Nanoparticulate films and clean water: Ni/Pt-Fe•TiUNP , silica scaffold
- Ice: single crystal, nucleation, and anti-icing
- Environment: ammonia-air interface
- Instrument development: SBW-M Nonlinear Interferometer
Nanoparticulate films and clean water
The clean water project targets organic contaminants, bacteria, and pharmaceuticals in drinking water. The UN estimates that four out of ten people suffer from water scarcity; importantly, polluted water can be deadly.
This project is based on the wonderful oxidation potential of titania (aka, the white coloration in tooth paste, skim milk, salad dressing…). With a strong oxidation potential, titania turns deadly organic pollutants into harmless CO2 and water using sunlight. The issue is that native titania is rather inefficient in water. By making the titania particles ultrasmall (less than two nanometers – or 10,000 times smaller than the finest human hair), doping with a very small amount of iron, and decorating each particle with a single Pt or Au atom, the Shultz group succeeded in boosting the efficiency about 10 times – enough to clean water for a family of four in a four-hour sunlight day. Supported by the Department of Energy (DE-SC0020258), the group also aims to use these particles to selectively oxidize CO and reduce CO2.
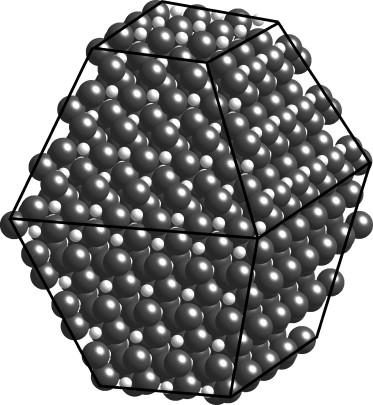
Titanium Ultra-nano Particles, referred as TiUNP, possess an exceedingly small size (<2nm), ensuring a high material utilization rate and a large surface adsorption area. Their inherently hydrophilic nature significantly enhances their photocatalytic efficiency compared to commercial TiO2 by an order of magnitude. Previous studies have demonstrated that doping these particles with iron and decorate with platinum at single-atom level leads to a further enhancement in organic degradation efficiency. Presently, we have developed the capability to incorporate a full spectrum of 4th-row transition metal ions into TiUNP, enabling precise tailoring of the photocatalyst properties to suit diverse tasks.

When working with TiUNP, an aqueous environment is essential. Owing to its hydrophilic nature and ultra-small size, the particles readily form a homogeneous solution in water. However, the filtration of such minuscule particles incurs significant costs. Therefore, our current approach aims to circumvent filtration by immobilizing these particles on a silica substrate or scaffold. There are opportunities for collaboration in this endeavor, and success could potentially save thousands of children daily, according to UN statistics.
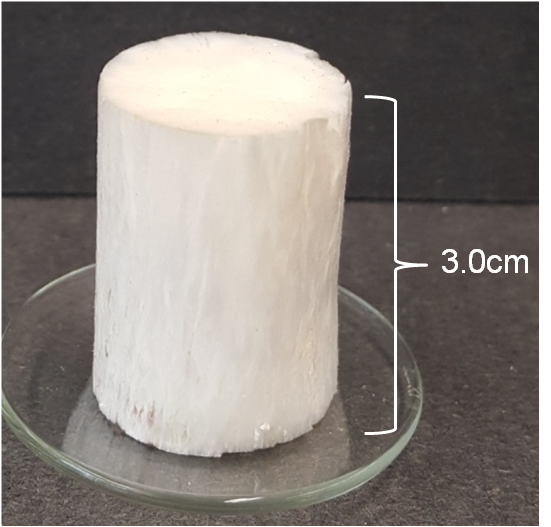
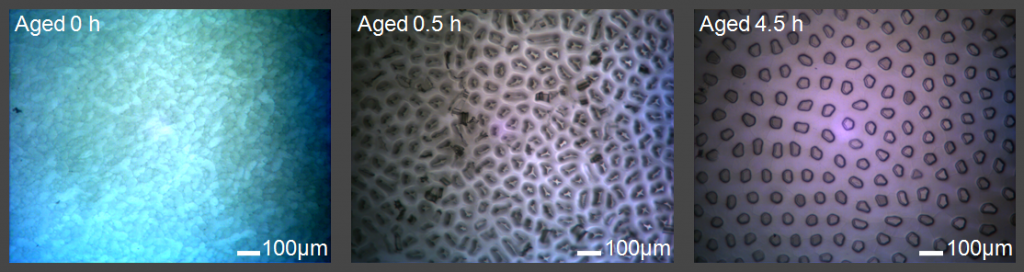
Ice: single crystal, nucleation, and anti-icing
The Shultz group has developed a technique to grow high-quality single crystal ice based on a modified Bridgeman apparatus. Targeted ice faces can be cut from the ice boules based on the orientation of the optical axis of the crystal. Being able to produce high-quality single crystals is necessary for obtaining reliable SFG spectra of different ice faces and is useful for future research involving ice.
The Shultz group is part of a Multidisciplinary University Research Initiative (MURI) that aims at understanding ice nucleation and anti-icing. The main goals for this project are to characterize hydration water at ice-binding sites, to develop multiscale modeling processes to account for antifreeze and ice recrystallization inhibition processes, to discover how biological and organic molecules nucleate ice, and to develop new techniques capable of simulating and probing buried ice-water interfaces. The Shultz group fits into this effort with experience of taking linear and nonlinear vibrational spectroscopic measurements, which will be used to better understand the interaction of water molecules with molecules that either inhibit or promote ice growth. In order to probe the buried ice-water interface, the new technique of Phase-Sensitive Reflection Coherent Anti-Stokes Raman Spectroscopy (PSR-CARS) will be developed. This technique will use CARS with the nonlinear interferometer that has been previously developed in the Shultz lab in order to obtain phase-sensitive measurements that are interface-specific. Opportunities exist for new students to become involved in this project.
Environment: ammonia
The current environmental project focuses on ammonia. The ability to turn molecular nitrogen into ammonia (fertilizer) has been characterized as the single most important chemical discovery ever: it is responsible for half the nitrogen atoms in every person’s proteins! Unfortunately, half of all fertilizer escapes, polluting water (think bright green algae) or finds its way into the atmosphere. Ammonia’s fate in the atmosphere is largely unknown. What is known is that ammonia is the only significant concentration basic gas in the atmosphere. As a base, it reacts with the plethora of acidic gases to form solids known as cloud condensation nuclei. Clouds, in turn, are the largest uncertainty for understanding the environment. This project focuses on interaction between aqueous solutions and ice with ammonia. The ultimate goal from understanding these interactions is to design fertilizers that hold ammonia in the soil to be accessible to the plants it was put there to fertilize. Current opportunities focus on characterizing the fundamental interactions, supported by NSF Award # 1807913
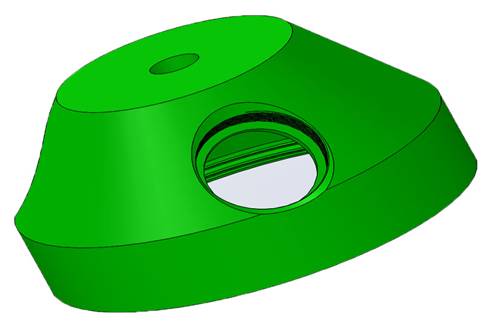

Instrument development
Whether developing the photocatalyst scaffold or investigating ammonia-solution Whether developing the photocatalyst scaffold or investigating ammonia-solution interactions, we require suitable instrumentation. Although numerous tools exist for probing solid – especially metal – surfaces, tools for probing complex, flexible, high vapor pressure interfaces are just being developed. The Shultz group invented one: a nonlinear interferometer. Since any surface contains seven to ten orders of magnitude fewer molecules than the bulk phases on either side of the interface, the first requirement is great sensitivity to the surface. For complex mixtures, the second requirement is being able to distinguish among the multiple surface species. The Shultz group uses a technique called sum frequency generation (SFG) to provide the surface sensitivity. Distinguishing among multiple surface species and measuring their concentration requires measuring a complex (in the mathematical sense) signal. We invented a nonlinear interferometer to measure the complex signal. Our current target is to extend this instrument to measure small differences as the surface molecules react to generate new materials. Materials that interact on ice surfaces likely change the surface morphology – either roughening or leveling it. The target of the not-yet-existing Nomariscope is to detect the morphology changes. We are now having three nonlinear systems for different research needs:1) HAL system, which has successfully measured solid surfaces. 2)Diana system, which has successfully measured solid and about to realize the expansion to liquid sample. 3)Zeus system, an advanced femto-second system which is under construction. Opportunities exist to get involved with creating instruments that enable discoveries well beyond the Shultz laboratory.
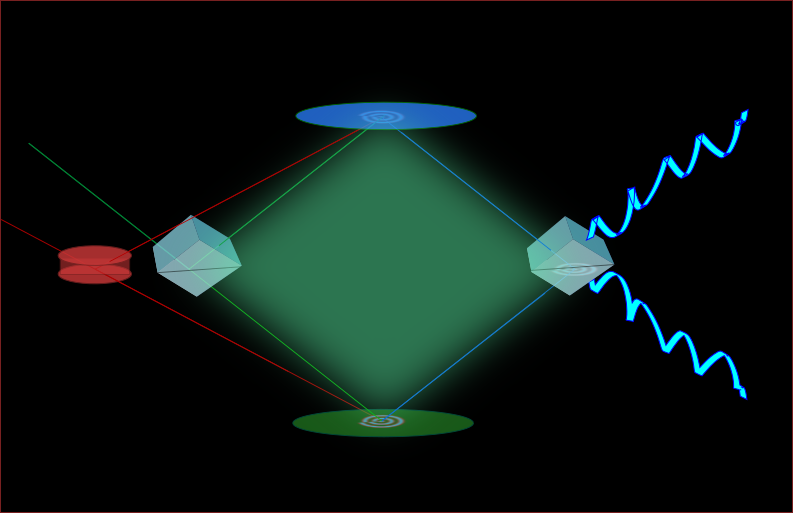